Pulsed Dielectric Barrier Discharges for Gas-Phase Composition Control: A Simulation Model
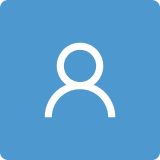
Round 1
Reviewer 1 Report
Comments and Suggestions for AuthorsRuggero Barni, et al. studied the controlling of the gas-phase composition using pulsed dielectric barrier discharges. Basically, this is a good study.
I have the following suggestions for a minor revision.
The whole study is a simulation work. The authors need to emphasize this information in the title.
The authors need to compare the calculated results with published experimental results.
Author Response
First referee comments
R1) Ruggero Barni, et al. studied the controlling of the gas-phase composition using pulsed dielectric barrier discharges. Basically, this is a good study. I have the following suggestions for a minor revision.
The whole study is a simulation work. The authors need to emphasize this information in the title.
A1) We have slightly modified the title.
New Title: “Pulsed Dielectric Barrier Discharges for the gas-phase composition control: a simulation model”
R2) The authors need to compare the calculated results with published experimental results.
A2) We have added a few sentences reporting experimental findings, to put our results in the contest.
We also increase the bibliography to have a more throughout vision of the state of the art.
New line 79-95) Many results about DBD in air have been collected both from the experimental and from the theoretical point of view. About the latter, the simulation of the chemical and physical phenomena characterizing DBD plasmas in air is not trivial. Three main methods have been applied to simulate non-thermal plasmas [12]: the kinetic approach [13], the fluid one [14-15] and some global models [16]. Ozone production in a surface DBD was studied by using a simple parametric model in [17]. The model was validated against some experimental ozone density measurements. They also observe ozone de-pletion by quenching reactions at high power level. Another paper studied the chemical species concentration produced in a Surface DBD reactor, in humid air. The simulation comprises more than 600 reaction paths [18]. Another parametric model [19], based on a limited reaction paths of 36 reactions was used to study the interplay between O3 and NOx evolutions, this in a plasma actuator device. Another approach proposed a two-stage model, including the discharge and the afterglow to study the main plasma chemistry processes of a atmospheric pressure air plasmas including 103 reaction paths [20]. Modeling of nanosecond pulse discharges in atmospheric pressure mixtures are focused mainly on the very short timescales of the discharge development and its effects for instance in energy applications [21].
New “bibliography”) X. Alves, L.; Bogaerts, A.; Guerra, V.; Turner, M. Foundations of modelling of nonequilibrium low-temperature plasmas. Plasma Sources Sci. Technol. 2018, 27, 023002.
- Donkó, Z. Particle simulation methods for studies of low-pressure plasma sources. Plasma Sources Sci. Technol. 2011, 20, 024001.
- Cristofolini, A.; Popoli, A. A multi-stage approach for DBD modelling. J. Phys.: Conf. Ser. 2019, 1243, 012012.
- Cristofolini, A.; Popoli, A.; Neretti, G. A multi-stage model for dielectric barrier discharge in atmospheric pressure air. Int. J. Appl. Electromagn. Mech. 2020, 63, S21–S29.
X, Hurlbatt, A.; Gibson, A.R.; Schröter, S.; Bredin, J.; Foote, A.P. S.; Grondein, P.; O’Connell, D.; Gans, T. Concepts, capabilities and limitations of global models: a review. Plasma Process. Polym. 2017, 14 1600138.
- Sakiyama, Y.; Graves, D.B.; Chang, H-W.; Shimizu, T.; Morfill, G.E. Plasma chemistry model of surface microdischarge in humid air and dynamics of reactive neutral species. J. Phys. D: Appl. Phys. 2012, 45, 425201.
- Shimizu, T.; Sakiyama, Y.; Graves, D.B.; Zimmermann, J.L.; Morfill, G.E. The dynamics of ozone generation and mode transition in air surface micro-discharge plasma at atmospheric pressure. New J. Phys. 2012, 14, 103028.
- Park, S.; Choe, W.; Jo, C. Interplay among ozone and nitrogen oxides in air plasmas: rapid change in plasma chemistry. Chem. Eng. J. 2018, 352, 1014–21.
- Pierotti, G.; Piferi, C.; Popoli, A.; Cavedon, M.; Cristofolini, A.; Martines, E.; Riccardi, C. A novel two-stage kinetic model for surface DBD simulations in air. Plasma Sources Science and Technology 2023, 32, XXX
- Pourali, N.; Hessel, V.; Rebrov, E.V. The Effects of Pulse Shape on the Selectivity and Production Rate in Non-oxidative Coupling of Methane by a Micro-DBD Reactor. Plasma Chem Plasma Process 2022, 42, 619–640.
Author Response File: Author Response.pdf
Reviewer 2 Report
Comments and Suggestions for AuthorsAtmospheric-pressure dielectric barrier discharges (DBD) are quite promising for a number of applications, from plasma-chemical synthesis to surface treatment. In particular, DBD driven by repetitive nanosecond pulses is very efficient and flexible in production of the desired chemical species. In this work, the authors describe a simplified model of such discharges that is then used to optimize the voltage waveform in order to maximize the production of certain species.
The model is quite reasonable, and the results are credible and potentially quite useful. The paper is also written clearly.
Prior to publication, the authors should address the following issue. It appears that they assume that as a pulse is applied to the DBD, each point in the discharges experiences the same action of the pulsed electric field. However, a pulse can typically produce just a few filaments where the ionization and chemistry take place, and the next pulse can go through a different region of the discharge. So, on average, the entire volume will experience ionization and chemistry, but for a given point, only a part of the overall pulse train will be experienced. Please clarify this and insert arguments that support the model used in this work.
Author Response
Second referee comments
Atmospheric-pressure dielectric barrier discharges (DBD) are quite promising for a number of applications, from plasma-chemical synthesis to surface treatment. In particular, DBD driven by repetitive nanosecond pulses is very efficient and flexible in production of the desired chemical species. In this work, the authors describe a simplified model of such discharges that is then used to optimize the voltage waveform in order to maximize the production of certain species.
The model is quite reasonable, and the results are credible and potentially quite useful. The paper is also written clearly. Prior to publication, the authors should address the following issue.
R3) It appears that they assume that as a pulse is applied to the DBD, each point in the discharges experiences the same action of the pulsed electric field. However, a pulse can typically produce just a few filaments where the ionization and chemistry take place, and the next pulse can go through a different region of the discharge. So, on average, the entire volume will experience ionization and chemistry, but for a given point, only a part of the overall pulse train will be experienced. Please clarify this and insert arguments that support the model used in this work.
A3) We have added a sentence to discuss the effect of filaments happening at different locations and times. We discussed it in the paragraph at lines 319-329.
New line 350-354) “In any way, when considering a specific location in the discharge gap, it is possible that no plasma filaments pass through it, on some of the cycles. Although addressing this requires a dedicated model and suitable experimental informations, we think that the discussion about the effects of a duty-cycle, that we now undertake, can shed light even on this more complicated situations.”
Author Response File: Author Response.pdf
Reviewer 3 Report
Comments and Suggestions for AuthorsThe paper ‘Control of the gas-phase composition (of atmospheric pressure air plasmas) using pulsed Dielectric Barrier Discharges’ deals with a numerical campaign caried out to evaluate plasma chemistry in air with different supplying voltage strategies and gas composition. A nanosecond pulse has been adopted to ignite the discharge with a repetition rate of 40 kHz (25 us period). Different duty cycles on/off have been used to compare the production efficiency of reactive species (especially ozone). The presence of humidity, nitrogen oxide and ammonia has been considered in main plasma kinetic processes. An application of the presented model to a ‘real life’ reactor is showed at the end of the paper.
It is my opinion that the manuscript presents an interesting approach, showing results useful to understand in a deeper way plasma kinetic in atmospheric pressure air. Despite this, there are several issues that must be fixed before the publication. For this reason, I suggest a Major Revision.
Main issues are listed below
- Title should be modified avoiding the use of parenthesis.
- English must be carefully checked. Just as an example
o Line 9 and 10 ‘Control or at least prediction of the gas-phase composition in atmospheric pressure cold plasmas is key to evaluate the potential of plasma treatments…’. What does it means that gas-phase composition ‘is key’? Is a key parameter? It plays a key role?
o Line 13 ‘We presents’, it should be we present.
o Line 244 ‘Metastable, weakly interacting states remain in the discharge gas-phase’. This sentence is not clear, should be rephrased.
o Line 246 ‘The last one is removed by diffusion together all the other discharge products’. Is it ‘together with’?
o Line 274 ‘This condition roughly correspond’. It should be ‘corresponds’.
o Others…
- Line 149. In their simulation authors are fixing a starting electron density of 1.2 10^14 cm-3 and electron temperature of 4 eV (Line 196). As an example, in reference ‘Brisset Plasma Sources Science and Technology, Volume 32, Number 6’ an average electron density of 10^15 and an electron temperature of 3 eV has been evaluated in a uniform nanosecond discharge (the ‘same’ authors are modelling). Authors should give some information about relations between inputs and outputs in their simulation.
- Authors utilize a Maxwellian distribution for the electron energy. Even though this could be a good approximation during the application of the electric field (i.e. 2 ns discharge), it is well known that in air, this distribution is not Maxwellian at all. Authors should discuss this assumption because their model is used mostly in the afterglow (25 us with respect 2 ns).
- Lines 256-257: Why a nanosecond discharge with a 40 kHz repetition rate corresponds to a sinusoidal discharge with a 20 kHz frequency?
- Why are authors using a 40 kHz repetition rate?
- All the figures showing species concentrations are hard to understand. Colors are confusing. In figures 6 and 7 there are different species with the same color. It is not possible to understand which species is which line.
- In figure 2, why electron density is not increasing at 10^14 during pulses following the first one?
- In fig. 6 and 7 there are species that, when the discharge is off, decrease with steps. Why are these steps presents? In these figures pulses are given with 5 on / 5 off and 2 on and 8 off. Why not 3 on and 8 off (as an example)? Why not other values?
- In the section 3.5 the model is used to simulate a real-life discharge. This discharge is a surface DBD discharge. A surface discharge strongly differ from a volumetric one. The electric filed is strongly non-homogeneous and also the plasma filament present strong non-homogeneity. What about electron density and temperature? Are these values still the same?
Comments on the Quality of English LanguageEnglish must be improved. There are several grammar and sentence errors
Author Response
Third referee comments
The paper ‘Control of the gas-phase composition (of atmospheric pressure air plasmas) using pulsed Dielectric Barrier Discharges’ deals with a numerical campaign caried out to evaluate plasma chemistry in air with different supplying voltage strategies and gas composition. A nanosecond pulse has been adopted to ignite the discharge with a repetition rate of 40 kHz (25 us period). Different duty cycles on/off have been used to compare the production efficiency of reactive species (especially ozone). The presence of humidity, nitrogen oxide and ammonia has been considered in main plasma kinetic processes. An application of the presented model to a ‘real life’ reactor is showed at the end of the paper.
It is my opinion that the manuscript presents an interesting approach, showing results useful to understand in a deeper way plasma kinetic in atmospheric pressure air. Despite this, there are several issues that must be fixed before the publication. For this reason, I suggest a Major Revision.
Main issues are listed below
R4) Title should be modified avoiding the use of parenthesis.
A4) We have slightly modified the title, as stated in A1.
New Title: “Pulsed Dielectric Barrier Discharges for the gas-phase composition control: a simulation model”
R5) English must be carefully checked. Just as an example
o Line 9 and 10 ‘Control or at least prediction of the gas-phase composition in atmospheric pressure cold plasmas is key to evaluate the potential of plasma treatments…’. What does it means that gas-phase composition ‘is key’? Is a key parameter? It plays a key role?
o Line 13 ‘We presents’, it should be we present.
o Line 244 ‘Metastable, weakly interacting states remain in the discharge gas-phase’. This sentence is not clear, should be rephrased.
o Line 246 ‘The last one is removed by diffusion together all the other discharge products’. Is it ‘together with’?
o Line 274 ‘This condition roughly correspond’. It should be ‘corresponds’.
o Others…
A5) Thank you for the remarks and sorry for the many typos, that we try to correct and also to im prove the language and grammar
New lines 9-10) “Control or at least prediction of the gas-phase composition in atmospheric pressure cold plasmas is very important to evaluate the potential of plasma treatments”
New lines 266-268)” Metastable states (narrowly excited states that cannot radiatively decays, because they have only forbidden transitions, which make their lifetimes much longer) and other weakly interacting states remain in the discharge gas-phase.”
New line 270-271) “In the end also they are removed by diffusion together with all the other stable discharge products.”
R6) Line 149. In their simulation authors are fixing a starting electron density of 1.2 10^14 cm-3 and electron temperature of 4 eV (Line 196). As an example, in reference ‘Brisset Plasma Sources Science and Technology, Volume 32, Number 6’ an average electron density of 10^15 and an electron temperature of 3 eV has been evaluated in a uniform nanosecond discharge (the ‘same’ authors are modelling). Authors should give some information about relations between inputs and outputs in their simulation.
A6) Thank you for bringing attention to this recent paper, that we added to the bibliography. The values quoted refers to a helium+water vapour discharge, so we believe that our, not so different, values are a better reference choice, being based on the referred measurements in our past experiments.
We discussed also the effect of the electron density on the chemical kinetics evolution of discharges in our previous paper (see ref. [8]/[9]). We added a sentence to discuss this point.
New lines 166-168) “This value is also in the same range of other reported results [X1]. A discussion of the effect of the electron density on the chemical kinetics of the discharges can be found in our previous works [8,9].”
New ref) Brisset, A.; Bieniek, M.; Invernizzi, L.; Hasan, M.; Walsh, J., Niemi, K.; Wagenaars, E. The formation of O and H radicals in a pulsed discharge in atmospheric pressure helium with water vapour admixtures. Plasma Sources Sci. Technol. 2023, 32, 065004.
R7) Authors utilize a Maxwellian distribution for the electron energy. Even though this could be a good approximation during the application of the electric field (i.e. 2 ns discharge), it is well known that in air, this distribution is not Maxwellian at all. Authors should discuss this assumption because their model is used mostly in the afterglow (25 us with respect 2 ns).
A7) We have added a sentence to explain better this point. In our strictly drift approximation scheme, electrons energy is controlled by the electric field in the ionizing wave. In the afterglow, the electric field is much smaller (see for instance ref.12) and electron energy drops to near the set temperature.
Electrons are also quickly removed so their effect on the afterglow evolution is minor. It is during the ionizing wave passing that their energy is relevant and referee agrees that a Maxwellian could be a reasonable approximation. We discussed also the effect of the electron temperature on the chemical kinetics evolution of discharges in our previous paper (see ref. [8]/[9]).
New line 214-217) In the afterglow, after the ionizing wave passage, the electric field is much smaller [22] and so the energy of the electrons, which was approximated by the set temperature [8]. A discussion of the effect of the electron temperature during the discharge pulse on the chemical kinetics can be found in our previous works [8,9].
R8) Lines 256-257: Why a nanosecond discharge with a 40 kHz repetition rate corresponds to a sinusoidal discharge with a 20 kHz frequency?
A8) We have added a sentence to explain this point.
New line 280-282) “These conditions correspond to a symmetric DBD fed by a supply with a 20 kHz sinusoidal HV signal, where the discharges happen and repeat in each half-cycle.”
R9) Why are authors using a 40 kHz repetition rate?
A9) We have added a sentence to explain this point.
New line 282-285) “This broadly corresponds to the experimental conditions we have investigated previously [14,16]. We discussed the effect of the repetition frequency on the chemical kinetics evolution of discharges in our previous papers [9].”
R10) All the figures showing species concentrations are hard to understand. Colors are confusing. In figures 6 and 7 there are different species with the same color. It is not possible to understand which species is which line.
A10) We added a few label to the curves of the relevant species discussed in the text. We also point out that the graphs are on a log-log scale, so the concentration of many minority species is really small.
We could modify the picture layout as suggested by the editing team, if this could help the readers.
R11) In figure 2, why electron density is not increasing at 10^14 during pulses following the first one?
A11) In the text (line 255) we explained that “To ease comparison, only the densities calculated at the end of each discharge are reported on the graph at later times”, so you cannot see the peak electron density on the plot. We have added the sentence to the Caption, to help readers.
R12) In fig. 6 and 7 there are species that, when the discharge is off, decrease with steps. Why are these steps presents? In these figures pulses are given with 5 on / 5 off and 2 on and 8 off. Why not 3 on and 8 off (as an example)? Why not other values?
A12) In the text (line 255) we explained that “To ease comparison, only the densities calculated at the end of each discharge are reported on the graph at later times”, so you cannot see the evolution of the densities in the intermediate times. This produces the stepwise behaviour. The steps mark the sequence repetitions, with discharges either on or off. We added a sentence to the caption to explain to readers.
New Captions 6/7) “To ease comparison, only the densities calculated at the end of each repetition are reported on the graph at later times, producing the stepwise trends.”
R13) In the section 3.5 the model is used to simulate a real-life discharge. This discharge is a surface DBD discharge. A surface discharge strongly differ from a volumetric one. The electric filed is strongly non-homogeneous and also the plasma filament present strong non-homogeneity. What about electron density and temperature? Are these values still the same?
A13) We agree with the referee comment. However we believe that an approximate 0D model can still be useful as a guide. We added a comment to underline this point.
New line 503-504) “Within this approximation the transverse non-homogeneities in the electric field strength and in the plasma filament are not considered.”
Author Response File: Author Response.pdf
Round 2
Reviewer 3 Report
Comments and Suggestions for AuthorsAuthors made a significan improvement in paper quality. It is my opinion that the manuscript can be published in the present form