Application of the Integrated Supercritical Fluid Extraction–Impregnation Process (SFE-SSI) for Development of Materials with Antiviral Properties
Abstract
: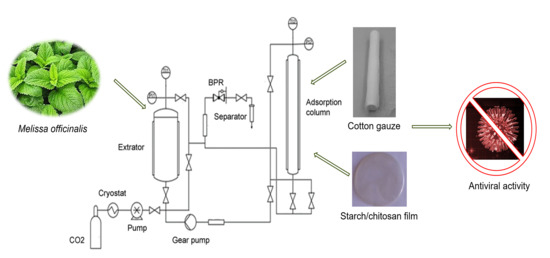
1. Introduction
2. Materials and Methods
2.1. Materials
2.2. Starch-Chitosan Film (SCF) Preparation
2.3. Supercritical Fluid Extraction (SFE)
2.3.1. Laboratory Scale Extraction
2.3.2. Pilot Scale Extraction
2.4. Integrated Supercritical Fluid Extraction–Impregnation Process (SFE-SSI)
2.5. Re-Extraction of the Impregnated Samples
2.6. Analytical Procedure
2.6.1. Gas Chromatography (GC) and Gas Chromatography/Mass Spectrometry (GC/MS)
2.6.2. High Performance Liquid Chromatography (HPLC)
2.7. FTIR Analysis
2.8. Antiviral Activity
3. Results and Discussion
3.1. Supercritical Fluid Extraction (SFE)
3.2. Integrated SFE-SSI Process
3.2.1. Influence of SFE-SSI Processing Mode on the Impregnation Yield of M. officinalis Extract into Cotton Gauze
3.2.2. Influence of Plant to Carrier Mass Ratio on the Impregnation Yield of M. officinalis Extract
3.3. Chemical Profile of Extracts and Re-Extracts
3.4. FTIR Analysis
3.5. Antiviral Activity
4. Conclusions
Author Contributions
Funding
Institutional Review Board Statement
Informed Consent Statement
Data Availability Statement
Acknowledgments
Conflicts of Interest
References
- Whitley, R.J.; Roizman, B. Herpes simplex virus infections. Lancet 2001, 357, 1513–1518. [Google Scholar] [CrossRef]
- Akhtar, J.; Shukla, D. Viral entry mechanisms: Cellular and viral mediators of herpes simplex virus entry. FEBS J. 2009, 276, 7228–7236. [Google Scholar] [CrossRef]
- Schnitzler, P.; Schuhmacher, A.; Astani, A.; Reichling, J. Melissa officinalis oil affects infectivity of enveloped herpesviruses. Phytomedicine 2008, 15, 734–740. [Google Scholar] [CrossRef]
- Allahverdiyev, A.; Duran, N.; Ozguven, M.; Koltas, S. Antiviral activity of the volatile oils of Melissa officinalis L. against Herpes simplex virus type-2. Phytomedicine 2004, 11, 657–661. [Google Scholar] [CrossRef]
- Khan, M.T.H.; Ather, A.; Thompson, K.D.; Gambari, R. Extracts and molecules from medicinal plants against herpes simplex viruse. Antivir. Res. 2005, 67, 107–119. [Google Scholar] [CrossRef]
- Mukhtar, M.; Arshad, M.; Ahmad, M.; Pomerantz, R.J.; Wigdahl, B.; Parveen, Z. Antiviral potential of medicinal plants. Virus Res. 2008, 131, 11–120. [Google Scholar] [CrossRef]
- Nolkemper, S.; Reichling, J.; Stintzing, F.C.; Carle, R.; Schnitzler, P. Antiviral effect of aqueous extracts from species of the Lamiaceae family against Herpes simplex virus type 1 and type 2 invitro. Planta Med. 2006, 72, 1378–1382. [Google Scholar] [CrossRef] [Green Version]
- Geuenich, S.; Goffinet, C.; Venzke, S.; Nolkemper, S.; Baumann, I.; Plinkert, P.; Reichling, J.; Keppler, O.T. Aqueous extracts from peppermint, sage and lemon balm leaves display potent anti-HIV-1 activity by increasing the virion density. Retrovirology 2008, 5, 27. [Google Scholar] [CrossRef] [Green Version]
- Carvalho, F.; Duarte, A.P.; Ferreira, S. Antimicrobial activity of Melissa officinalis and its potential use in food preservation. Food Biosci. 2021, 44, 101437. [Google Scholar] [CrossRef]
- Dimitrova, Z.; Dimov, B.; Manolova, N.; Pancheva, S.; Ilieva, D.; Shishkov, S. Antiherpes effect of Melissa officinalis L. extracts. Acta Microbiol. Bul. 1993, 29, 65–72. [Google Scholar]
- Koytchev, R.; Aiken, R.G.; Dundarov, S. Balm mint extract (Lo-701) for topical treatment of recurring Herpes labialis. Phytomedicine 1999, 6, 225–230. [Google Scholar] [CrossRef]
- Mazzanti, G.; Battinelli, L.; Pompeo, C.; Serrilli, A.M.; Rossi, R.; Sauzullo, I.; Mengoni, F.; Vullo, V. Inhibitory activity of Melissa officinalis L. extract on Herpes simplex virus type 2 replication. Nat. Prod. Res. 2008, 22, 1433–1440. [Google Scholar] [CrossRef] [PubMed]
- Milovanovic, S.; Stamenic, M.; Markovic, D.; Radetic, M.; Zizovic, I. Solubility of thymol in supercritical carbon dioxide and its impregnation on cotton gauze. J. Supercrit. Fluids 2013, 84, 173–181. [Google Scholar] [CrossRef]
- Jayakumar, R.; Prabaharan, M.; Sudheesh Kumar, P.T.; Nair, S.V.; Tamura, H. Biomaterials based on chitin and chitosan in wound dressing applications. Biotechnol. Adv. 2011, 29, 322–337. [Google Scholar] [CrossRef] [PubMed]
- Reverchon, E.; De Marco, I. Supercritical fluid extraction and fractionation of natural matter. J. Supercrit. Fluids 2006, 38, 146–166. [Google Scholar] [CrossRef]
- Kikic, I.; Vecchione, F. Supercritical impregnation of polymers. Curr. Opin. Solid State Mater. Sci. 2003, 7, 399–405. [Google Scholar] [CrossRef]
- Khaw, K.-Y.; Parat, M.-O.; Shaw, P.N.; Falconer, J.R. Solvent Supercritical Fluid Technologies to Extract Bioactive Compounds from Natural Sources: A Review. Molecules 2017, 22, 1186. [Google Scholar] [CrossRef]
- da Silva, R.P.F.F.; Rocha-Santos, T.A.P.; Duarte, A.C. Supercritical fluid extraction of bioactive compounds. TrAC-Trends Anal. Chem. 2016, 76, 40–51. [Google Scholar] [CrossRef] [Green Version]
- Sutil, G.A.; Andrade, K.S.; Rebelatto, E.A.; Lanza, M. Effects of incorporation of pure or multicomponent active agents in biopolymers for food packaging using supercritical CO2. Trends. Food Sci. Technol. 2022, 120, 349–362. [Google Scholar] [CrossRef]
- Champeau, M.; Thomassin, J.M.; Tassaing, T.; Jérôme, C. Drug loading of polymer implants by supercritical CO2 assisted impregnation: A review. J. Control. Release 2015, 209, 248–259. [Google Scholar] [CrossRef]
- Ivanovic, J.; Milovanovic, S.; Zizovic, I. Utilization of supercritical CO2 as a processing aid in setting functionality of starch-based materials. Starch-Stärke 2016, 68, 821–833. [Google Scholar] [CrossRef]
- Tutek, K.; Masek, A.; Kosmalska, A.; Cichosz, S. Application of Fluids in Supercritical Conditions in the Polymer Industry. Polymers 2021, 13, 729. [Google Scholar] [CrossRef] [PubMed]
- Goñi, M.L.; Gañán, N.A.; Martini, R.E. Supercritical CO2-assisted dyeing and functionalization of polymeric materials: A review of recent advances (2015–2020). J. CO2 Util. 2021, 54, 101760. [Google Scholar] [CrossRef]
- Varona, S.; Rodríguez-Rojo, S.; Martín, Á.; Cocero, M.J.; Duarte, C.M.M. Supercritical impregnation of lavandin (Lavandula hybrida) essential oil in modified starch. J. Supercrit. Fluids 2011, 58, 313–319. [Google Scholar] [CrossRef]
- Almeida, A.P.; Rodríguez-Rojo, S.; Serra, A.T.; Vila-Real, H.; Simplicio, A.L.; Delgadilho, I.; da Costa, S.B.; da Costa, L.B.; Nogueira, I.D.; Duarte, C.M.M. Microencapsulation of oregano essential oil in starch-based materials using supercritical fluid technology. Innov. Food Sci. Emerg. Technol. 2013, 20, 140–145. [Google Scholar] [CrossRef]
- de Souza, A.C.; Dias, A.M.A.; Sousa, H.C.; Tadini, C.C. Impregnation of cinnamaldehyde into cassava starch biocomposite films using supercritical fluid technology for the development of food active packaging. Carbohydr. Polym. 2014, 102, 830–837. [Google Scholar] [CrossRef] [Green Version]
- Medeiros, G.R.; Ferreira, S.R.S.; Carciofi, B.A.M. High pressure carbon dioxide for impregnation of clove essential oil in LLDPE films Innov. Food Sci. Emerg. Technol. 2017, 41, 206–215. [Google Scholar] [CrossRef]
- Bastante, C.C.; Silva, N.H.C.S.; Cardoso, L.C.; Serrano, C.M.; Martínez de la Ossa, E.J.; Freire, C.S.R.; Vilela, C. Biobased films of nanocellulose and mango leaf extract for active food packaging: Supercritical impregnation versus solvent casting. Food Hydrocoll. 2021, 117, 106709. [Google Scholar] [CrossRef]
- Liparoti, S.; Franco, P.; Pantani, R.; De Marco, I. Supercritical CO2 impregnation of caffeine in biopolymer films to produce anti-cellulite devices. J. Supercrit. Fluids 2022, 179, 105411. [Google Scholar] [CrossRef]
- Franco, P.; Incarnato, L.; De Marco, I. Supercritical CO2 impregnation of α-tocopherol into PET/PP films for active packaging applications. J. CO2 Util. 2019, 34, 266–273. [Google Scholar] [CrossRef]
- Fanovich, M.A.; Ivanovic, J.; Misic, D.; Alvarez, M.V.; Jaeger, P.; Zizovic, I.; Eggers, R. Development of polycaprolactone scaffold with antibacterial activity by an integrated supercritical extraction and impregnation process. J. Supercrit. Fluids 2013, 78, 42–53. [Google Scholar] [CrossRef]
- Fanovich, M.A.; Ivanovic, J.; Zizovic, I.; Misic, D.; Jaeger, P. Functionalization of polycaprolactone/hydroxyapatite scaffolds with Usnea lethariiformis extract by using supercritical CO2. Mater. Sci. Eng. C 2016, 58, 204–212. [Google Scholar] [CrossRef] [PubMed]
- Ivanovic, J.; Milovanovic, S.; Stamenic, M.; Fanovich, M.A.; Jaeger, P.; Zizovic, I. Application of an Integrated Supercritical Extraction and Impregnation Process for Incorporation of Thyme Extracts into Different Carriers. In Handbook on Supercritical Fluids, Fundamentals, Properties and Applications; Osborne, J., Ed.; Nova Science Publishers: New York, NY, USA, 2014; pp. 257–280. [Google Scholar]
- Zizovic, I.; Ivanovic, J.; Milovanovic, S.; Stamenic, M. Impregnations using supercritical carbon dioxide. In Supercritical CO2 Extraction and Its Applications; Roj, E., Ed.; Polish Foundations of the Opportunities Industrialization Centers “OIC Poland”: Lublin, Poland, 2014; pp. 23–34. [Google Scholar]
- Maksimović, S.; Tadić, V.; Ivanović, J.; Radmanović, T.; Milovanović, S.; Stanković, M.; Žižović, I. Utilization of the integrated process of supercritical extraction and impregnation for incorporation of Helichrysum italicum extract into corn starch xerogel. Chem. Ind. Chem. Eng. 2018, 24, 191–200. [Google Scholar] [CrossRef]
- Maksimović, S.; Tadić, V.; Zvezdanovic, J.; Zizovic, I. Utilization of supercritical CO2 in bioactive principles isolation from Helichrysum italicum and their adsorption on selected fabrics. J. Supercrit. Fluids 2021, 171, 105197. [Google Scholar] [CrossRef]
- Bogdanovic, A.; Tadic, V.; Arsic, I.; Milovanovic, S.; Petrovic, S.; Skala, D. Supercritical and high pressure subcritical fluid extraction from Lemon balm (Melissa officinalis L., Lamiaceae). J. Supercrit. Fluids 2016, 107, 234–242. [Google Scholar] [CrossRef]
- Marongiu, B.; Porcedda, S.; Piras, A.; Rosa, A.; Deiana, M.; Dessì, M.A. Antioxidant Activity of Supercritical Extract of Melissa officinalis Subsp. officinalis and Melissa officinalis Subsp. Inodora. Phytother. Res. 2004, 18, 789–792. [Google Scholar] [CrossRef]
- Rozzi, N.L.; Phippen, W.; Simon, J.E.; Singh, R.K. Supercritical Fluid Extraction of Essential Oil Components from Lemon-Scented Botanicals. LWT-Food Sci. Technol. 2002, 35, 319–324. [Google Scholar] [CrossRef]
- Angelov, G.; Penchev, P.; Condoret, J.S.; Camy, S. Optimizing the process of supercritical extraction of lemon balm (Melissa Officinalis L.). C. R. Acad. Bulg. Sci. 2010, 63, 1141–1146. [Google Scholar]
- Ribeiro, M.A.; Bernardo-Gil, M.G.; Esquível, M.M. Melissa officinalis, L.: Study of antioxidant activity in supercritical residues. J. Supercrit. Fluids 2001, 21, 51–60. [Google Scholar] [CrossRef]
- Pajnik, J.; Lukić, I.; Dikic, J.; Asanin, J.; Gordic, M.; Misic, D.; Zizovic, I.; Korzeniowska, M. Application of Supercritical Solvent Impregnation for Production of Zeolite Modified Starch-Chitosan Polymers with Antibacterial Properties. Molecules 2020, 25, 4717. [Google Scholar] [CrossRef]
- Vági, E.; Balázs, M.; Komoczi, A.; Mihalovits, M.; Székely, E. Fractionation of phytocannabinoids from industrial hemp residues with high-pressure technologies. J. Supercrit. Fluids 2020, 164, 104898. [Google Scholar] [CrossRef]
- Adams, R.P. Identification of Essential Oil Components by Gas Chromatography/Mass Spectrometry, 4th ed.; Allured Publishing Corporation: Carol Stream, IL, USA, 2007. [Google Scholar]
- Reed, L.; Muench, H. A simple method of estimating fifty percent endpoints. Am. J. Hyg. 1938, 27, 493–497. [Google Scholar]
- Shakeri, A.; Sahebkar, A.; Javadi, B. Melissa officinalis L.—A review of its traditional uses, phytochemistry and pharmacology. J. Etnopharm. 2016, 188, 204–228. [Google Scholar] [CrossRef] [PubMed]
- Jalal, Z.; El Atki, Y.; Lyoussi, B.; Abdellaoui, A. Phytochemistry of the essential oil of Melissa officinalis L. growing wild in Morocco: Preventive approach against nosocomial infections. Asian Pac. J. Trop. Biomed. 2015, 5, 458–461. [Google Scholar] [CrossRef] [Green Version]
- Khalili, G.; Mazloomifar, A.; Larijani, K.; Tehrani, M.S.; Azar, P.A. Solvent-free microwave extraction of essential oils from Thymus vulgaris L. and Melissa officinalis L. Ind. Crops Prod. 2018, 119, 214–217. [Google Scholar] [CrossRef]
- Prado, J.M.; Prado, G.H.C.; Meireles, M.A.A. Scale-up study of supercritical fluid extraction process for clove and sugarcane residue. J. Supercrit. Fluids 2011, 56, 231–237. [Google Scholar] [CrossRef] [Green Version]
- Belwal, T.; Chemat, F.; Venskutonis, P.R.; Cravotto, G.; Jaiswal, D.K.; Bhatt, I.D.; Devkota, H.P.; Luo, Z. Recent advances in scaling-up of non-conventional extraction techniques: Learning from successes and failures. TrAC-Trends Anal. Chem. 2020, 127, 115895. [Google Scholar] [CrossRef]
- Abdellatif, F.; Boudjella, H.; Zitouni, A.; Hassani, A. Chemical composition and antimicrobial activity of the essential oil from leaves of Algerian Melissa officinalis L. EXCLI J. 2014, 13, 772–781. [Google Scholar]
- Najafian, S. Storage conditions affect the essential oil composition of cultivated Balm Mint Herb (Lamiaceae) in Iran. Ind. Crops Prod. 2014, 52, 575–581. [Google Scholar] [CrossRef]
- Mimica-Dukic, N.; Bozin, B.; Sokovic, M.; Simin, N. Antimicrobial and Antioxidant Activities of Melissa officinalis L. (Lamiaceae) Essential Oil. J. Agric. Food Chem. 2004, 52, 2485–2489. [Google Scholar] [CrossRef]
- Abdel-Naime, W.A.; Fahim, J.R.; Fouad, M.A.; Kamel, M.S. Antibacterial, antifungal, and GC–MS studies of Melissa officinalis. S. Afr. J. Bot. 2019, 124, 228–234. [Google Scholar] [CrossRef]
- Basta, A.; Tzakou, O.; Couladis, M. Composition of the leaves essential oil of Melissa officinalis s. l. from Greece. Flavour Fragr. J. 2005, 20, 642–644. [Google Scholar] [CrossRef]
- Caniova, A.; Brandsteterova, E. HPLC analysis of phenolic acids in Melissa officinalis. J. Liq. Chromatogr. Relat. Technol. 2001, 24, 2647–2659. [Google Scholar] [CrossRef]
- Kittler, J.; Krüger, H.; Ulrich, D.; Zeiger, B.; Schütze, W.; Böttcher, C.; Krähmer, A.; Gudi, G.; Kästner, U.; Heuberger, X.; et al. Content and composition of essential oil and content of rosmarinic acid in lemon balm and balm genotypes (Melissa officinalis). Genet. Resour. Crop Evol. 2018, 65, 1517–1527. [Google Scholar] [CrossRef] [Green Version]
- Shekarchi, M.; Hajimehdipoor, H.; Saeidnia, S.; Gohari, A.R.; Hamedani, M.P. Comparative study of rosmarinic acid content in some plants of Labiatae family. Pharmacogn. Mag. 2012, 8, 37–41. [Google Scholar]
- Peev, G.; Penchev, P.; Penchev, D.; Angelov, G. Solvent extraction of rosemarinic acid from lemon balm and concentration of extracts by nanofiltration/Effect of plant pre-treatment by supercritical carbon dioxide. Chem. Eng. Res. Des. 2011, 89, 2236–2243. [Google Scholar] [CrossRef]
- Chung, C.; Lee, M.E.; Choe, K. Characterization of cotton fabric scouring by FT-IR ATR spectroscopy. Carbohydr. Polym. 2004, 58, 417–420. [Google Scholar] [CrossRef]
- Oh, S.Y.; Yoo, D.I.; Shin, Y.; Seo, G. FTIR analysis of cellulose treated with sodium hydroxide and carbon dioxide. Carbohydr. Res. 2005, 340, 417–428. [Google Scholar] [CrossRef]
- Bof, M.J.; Bordagaray, V.C.; Locaso, D.E.; García, M.A. Chitosan molecular weight effect on starch-compositefilm properties. Food Hydrocoll. 2015, 51, 281–294. [Google Scholar] [CrossRef]
- Ren, L.; Yan, X.; Zhou, J.; Tong, J.; Su, X. Influence of chitosan concentration on mechanical and barrier properties of corn starch/chitosan films. Int. J. Biol. Macromol. 2017, 105, 1636–1643. [Google Scholar] [CrossRef]
- Mathew, S.; Abraham, T.E. Characterization of ferulic acid incorporated starch–chitosan blend films. Food Hydrocoll. 2008, 22, 826–835. [Google Scholar] [CrossRef]
- Liu, H.; Adhikari, R.; Guo, Q.; Adhikari, B. Preparation and characterization of glycerol plasticized (high-amylose) starch–chitosan films. J. Food Eng. 2013, 116, 588–597. [Google Scholar] [CrossRef]
- Silva-Pereira, M.C.; Teixeira, J.A.; Pereira-Júnior, V.A.; Stefani, R. Chitosan/corn starch blend films with extract from Brassica oleraceae (red cabbage) as a visual indicator offish deterioration. LWT-Food Sci. Technol. 2015, 61, 258–262. [Google Scholar] [CrossRef] [Green Version]
- Dias, A.M.A.; Rey-Rico, A.; Oliveira, R.A.; Marceneiro, S.; Alvarez-Lorenzo, C.; Concheiro, A.; Júnior, R.N.C.; Braga, M.E.M.; de Sousa, H.C.J. Wound dressings loaded with an anti-inflammatory jucá (Libidibia ferrea) extract using supercritical carbon dioxide technology. J. Supercrit. Fluids 2013, 74, 34–45. [Google Scholar] [CrossRef] [Green Version]
- Astani, A.; Reichling, J.; Schnitzler, P. Melissa officinalis extract inhibits attachment of herpes simplex virus in vitro. Chemoterapy 2012, 58, 70–77. [Google Scholar] [CrossRef] [Green Version]
- Perumal, A.B.; Huang, L.; Nambiar, R.B.; He, Y.; Li, X.; Sellamuthu, P.S. Application of essential oils in packaging films for the preservation of fruits and vegetables: A review. Food Chem. 2021, 375, 131810. [Google Scholar] [CrossRef]
Cycle Number | Cycle Duration, h | Total Contact Time, h/min | mCO2/mp | mex.imp., mg | I, % | |
---|---|---|---|---|---|---|
Mode I | 1 | 5 | 5 | 5.94 | 6.3 | 0.34 ± 0.035 |
Mode II | 3 | 2 + 1 | 5/10 | 8.51 | 26.5 | 1.48 ± 0.127 |
Mode III | 5 | 1 | 5/20 | 10.62 | 44.6 | 2.24 ± 0.141 |
Mode IV | 7 | 1 | 7/30 | 12.63 | 16.2 | 0.91 ± 0.071 |
Carrier | mp/mc | I, % |
---|---|---|
Cotton gauze | 10 | 1.05 ± 0.092 |
25 | 2.24 ± 0.141 | |
50 | 2.27 ± 0.205 | |
SCF | 72 (1 film) | 8.71 ± 1.372 |
33 (2 films) | 5.44 ± 0.622 |
Compound | KI | SFE | Re-Extracted from Cotton Gauze | Re-Extracted from SCF | |
---|---|---|---|---|---|
1. | hexanoic acid | 975 | 0.42 | 0.55 | / |
2. | 3E-octen-2-ol | 982 | 0.21 | 0.23 | / |
3. | citronellal | 1151 | 0.08 | 0.58 | / |
4. | octanoic acid | 1152 | t | 0.97 | 0.09 |
5. | neomenthol | 1161 | 0.2 | / | / |
6. | 1-decen-3-ol | 1177 | 0.13 | t | t |
7. | neral | 1235 | 0.36 | 0.46 | 0.07 |
8. | geraniol | 1249 | 0.14 | 0.23 | t |
9. | geranial | 1268 | 0.57 | 2.57 | 0.13 |
10. | carvacrol | 1298 | 0.19 | t | 0.13 |
11. | menthyl acetate | 1302 | t | t | 0.2 |
12. | methyl geranate | 1322 | t | 0.33 | 0.3 |
13. | neryl acetate | 1353 | 0.29 | 2.99 | t |
14. | geranyl acetate | 1358 | 0.31 | / | / |
15. | tetradecene | 1391 | 0.13 | / | / |
16. | β-caryophyllene | 1417 | 3.52 | 0.34 | t |
17. | β-(E)-farnesene | 1452 | 0.13 | 0.33 | t |
18. | α-humulene | 1472 | 0.25 | / | / |
19. | menthyl lactate | 1473 | t | 0.44 | T |
20. | β-ionone | 1500 | 0.13 | / | / |
21. | germacrene D | 1503 | 0.74 | 0.24 | t |
22. | δ-cadinene | 1524 | t | 0.24 | 0.56 |
23. | dihydroactinolide | 1535 | 0.45 | 0.44 | t |
24. | nerolidol | 1554 | 0.08 | / | t |
25. | germacren D-4-ol | 1571 | 0.4 | 0.41 | 0.1 |
26. | ledol | 1588 | 0.08 | / | / |
27. | caryophyllene oxide | 1595 | 2.58 | / | 1.56 |
28. | humulene epoxide II | 1610 | 0.15 | 0.39 | 0.08 |
29. | 1-epi-cubenol | 1627 | 0.19 | 0.25 | 0.12 |
30. | α-cadinol | 1643 | 0.08 | 1.25 | / |
31. | caryophylla-4(12),8(13)-dien-5-β-ol | 1661 | 0.16 | 0.39 | 0.18 |
32. | 14-Hydroxy-(E)-caryophyllene | 1667 | 0.29 | / | 0.32 |
33. | methyl tetradecanoate | 1710 | 0.17 | 0.62 | 0.44 |
34. | amorpha-4,9-diene-2-ol | 1713 | t | 0.61 | 0.17 |
35. | 14-Hydroxy-α-muurolene | 1756 | 0.18 | / | / |
36. | oplopanone | 1745 | 0.3 | 2.2 | 0.55 |
37. | isolongifolol | 1781 | 0.22 | 0.45 | 0.41 |
38. | geranylcyclopentanone | 1811 | 0.11 | 0.56 | 0.33 |
39. | α-chenopodiol | 1855 | 0.3 | 0.39 | 0.28 |
40. | 1-hexadecanol | 1882 | 0.31 | 0.8 | 1.66 |
41. | nonadecane | 1900 | 0.2 | 0.87 | 0.61 |
42. | isophytol | 1943 | 0.11 | 1.28 | 0.41 |
43. | hexadecanoic acid | 1968 | 6.1 | 10.87 | 4.01 |
44. | geranyl linalool | 2031 | 3.03 | 1.76 | 0.14 |
45. | (6E,10E)-pseudophytol | 2058 | 0.71 | 1.55 | 0.61 |
46. | octadecanol | 2099 | 0.17 | 0.34 | 0.12 |
47. | (9Z,12Z)-octadecadienoic acid | 2134 | 26.11 | 0.98 | 1.02 |
48. | docosane | 2200 | 6.33 | 11.51 | 19.5 |
49. | 7α-hydroxy manool | 2237 | 0.53 | 0.35 | 0.33 |
50. | isopimpellin | 2250 | 0.88 | / | / |
51. | 4-epi-dehydro abietol | 2320 | 0.11 | / | / |
52. | trans-ferruginol | 2335 | 0.11 | 0.87 | 0.25 |
53. | tetracosane | 2400 | 0.88 | 0.99 | 2.71 |
54. | hexacosane | 2600 | 1.32 | 0.67 | 5.85 |
55. | heptacosane | 2700 | 5.71 | 6.97 | 9.28 |
56. | octacosane | 2800 | 0.31 | 5.87 | 0.44 |
57. | squalene | 2835 | 3.63 | 0.42 | 0.97 |
58. | nonacosane | 2900 | 6.33 | 7.9 | 21.35 |
59. | dotriacontane | 3200 | 2.21 | 1.21 | 1.31 |
60. | triacontane | 3000 | 5.67 | 4.46 | 11.91 |
61. | hentriacontane | 3100 | 4.89 | 8.51 | 2.15 |
62. | tocopherol | 3149 | 0.69 | / | / |
63. | β-sitosterol | 3187 | 0.65 | 1.71 | 0.86 |
64. | ergosterol | 3152 | 0.68 | 0.64 | 0.9 |
65. | dihydroergosterol | 3175 | 0.37 | 0.58 | 0.13 |
66. | stigmasterol | 3248 | 1.32 | 3.58 | 2.21 |
67. | (3β,22E)-ergosta-5,7,22-tren-3-ol acetate | 3269 | 0.61 | / | / |
68. | tritriacontane | 3300 | 4.97 | 4.12 | 2.35 |
69. | γ-sitosterol | 3351 | 0.68 | 1.78 | 1.81 |
Monoterpenoids | 2.72 | 8.04 | 0.83 | ||
Sesquiterpenoids | 9.76 | 8.05 | 4.66 | ||
Diterpenoids/triterpenoids | 8.92 | 6.23 | 2.71 | ||
Fatty acids and esters | 32.80 | 13.99 | 5.56 | ||
Hydrocarbons | 38.95 | 53.08 | 77.46 | ||
Phytosterols | 4.31 | 8.29 | 5.91 | ||
Other compounds | 1.70 | 1.37 | 1.78 | ||
Total | 99.16 | 99.05 | 98.91 |
Sample | Rosmarinic Acid, mg/gextract |
---|---|
SFE | 0.307 ± 0.006 |
Re-extracted from cotton gauze | 0.291 ± 0.006 |
Re-extracted from SCF | 0.017 ± 0.000 |
Publisher’s Note: MDPI stays neutral with regard to jurisdictional claims in published maps and institutional affiliations. |
© 2022 by the authors. Licensee MDPI, Basel, Switzerland. This article is an open access article distributed under the terms and conditions of the Creative Commons Attribution (CC BY) license (https://creativecommons.org/licenses/by/4.0/).
Share and Cite
Lukic, I.; Pajnik, J.; Nisavic, J.; Tadic, V.; Vági, E.; Szekely, E.; Zizovic, I. Application of the Integrated Supercritical Fluid Extraction–Impregnation Process (SFE-SSI) for Development of Materials with Antiviral Properties. Processes 2022, 10, 680. https://doi.org/10.3390/pr10040680
Lukic I, Pajnik J, Nisavic J, Tadic V, Vági E, Szekely E, Zizovic I. Application of the Integrated Supercritical Fluid Extraction–Impregnation Process (SFE-SSI) for Development of Materials with Antiviral Properties. Processes. 2022; 10(4):680. https://doi.org/10.3390/pr10040680
Chicago/Turabian StyleLukic, Ivana, Jelena Pajnik, Jakov Nisavic, Vanja Tadic, Erika Vági, Edit Szekely, and Irena Zizovic. 2022. "Application of the Integrated Supercritical Fluid Extraction–Impregnation Process (SFE-SSI) for Development of Materials with Antiviral Properties" Processes 10, no. 4: 680. https://doi.org/10.3390/pr10040680
APA StyleLukic, I., Pajnik, J., Nisavic, J., Tadic, V., Vági, E., Szekely, E., & Zizovic, I. (2022). Application of the Integrated Supercritical Fluid Extraction–Impregnation Process (SFE-SSI) for Development of Materials with Antiviral Properties. Processes, 10(4), 680. https://doi.org/10.3390/pr10040680